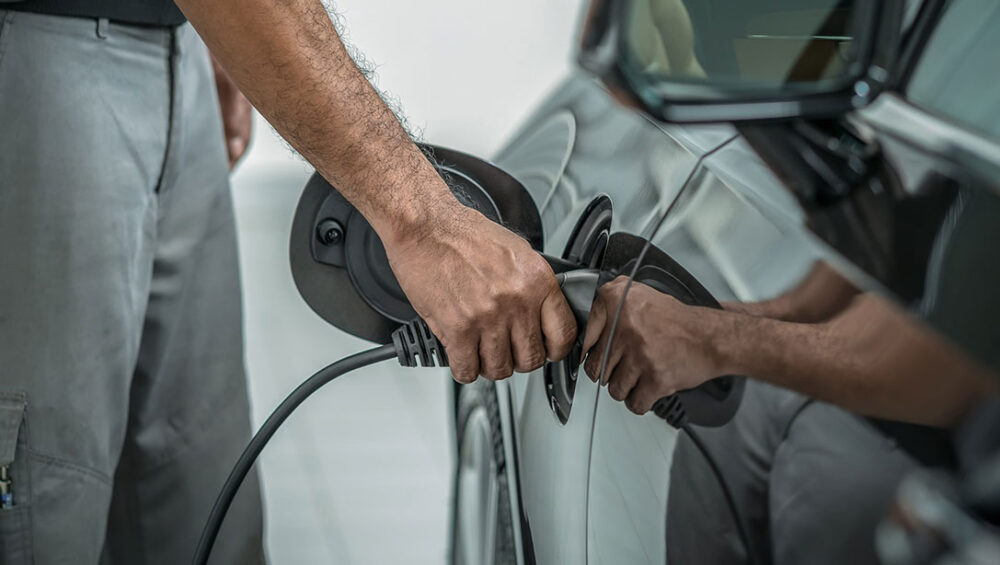
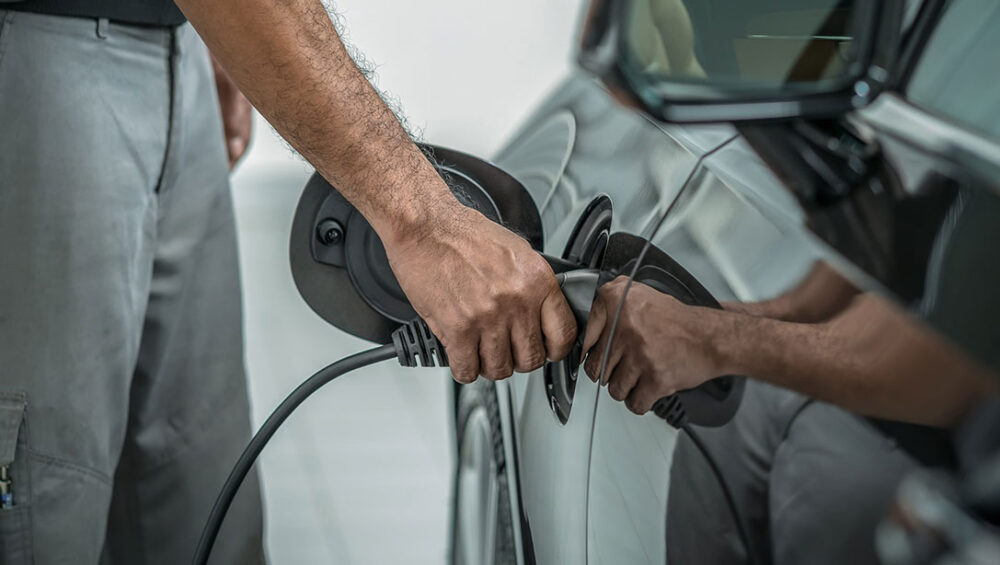
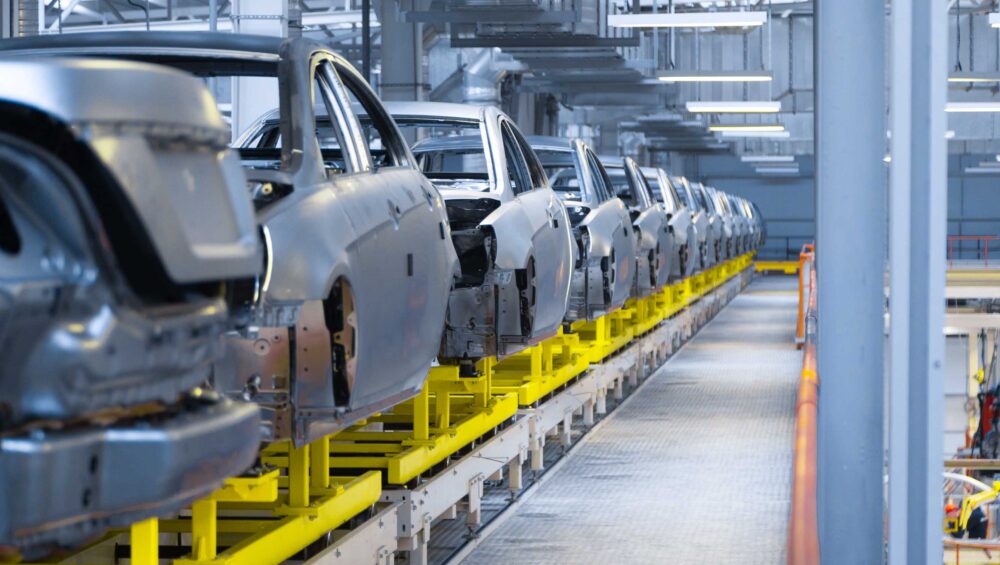
Building a Resilient Supply Chain: How EV Startups Can Boost Domestic Li-ion Cell Manufacturing
Building a Resilient Supply Chain: How EV Startups Can Boost Domestic Li-ion Cell Manufacturing
Li-ion cells are powering the world’s transition to EVs, and all countries, including India, are competing fiercely to reap maximum benefits from this transition by localising the supply chain.

Robin George
Vice President, Battery Advancement
Sources of Information:
- https://www.axios.com/2019/05/13/carbon-dioxide-concentration-earth-all-time-high
- https://climate.nasa.gov/climate_resources/7/graphic-carbon-dioxide-hits-new-high/
- https://newsroom.accenture.com/news/nearly-all-companies-will-miss-net-zero-goals-without-at-least-doubling-rate-of-carbon-emissions-reductions-by-2030-accenture-report-finds.htm#:~:text=1%2C%202022%20%E2%80%93%20While%20more%20than,Accenture%20(NYSE%3A%20ACN).
- https://www.eqmagpro.com/net-zero-many-india-inc-majors-could-be-net-zero-by-2050/#:~:text=More%20than%2030%20Indian%20companies,emissions%20by%202050%20or%20sooner.
- https://economictimes.indiatimes.com/news/international/world-news/only-29-of-the-top-50-firms-have-time-bound-carbon-neutrality-targets-report/articleshow/90916911.cms
- https://www.aboutamazon.com/news/sustainability/20-more-companies-from-around-the-globe-join-the-climate-pledge
- https://timesofindia.indiatimes.com/business/india-business/24-companies-including-tata-ril-pledge-to-be-carbon-neutral/articleshow/79072359.cms
- https://www.epa.gov/climateleadership/scope-1-and-scope-2-inventory-guidance#:~:text=Scope%201%20emissions%20are%20direct,boilers%2C%20furnaces%2C%20vehicles).
- https://economictimes.indiatimes.com/industry/renewables/cipla-adds-capacity-of-captive-renewable-energy-power-plant-in-maharashtra-karnataka/articleshow/91955693.cms?utm_source=contentofinterest&utm_medium=text&utm_campaign=cppst
- https://gailonline.com/RenewableEnergy.html
- https://renewablewatch.in/2022/09/13/hindalco-commissions-25-mw-captive-solar-plant-in-madhya-pradesh/
- https://www.electricalindia.in/hindustan-zincs-captive-power-plants-win-award/
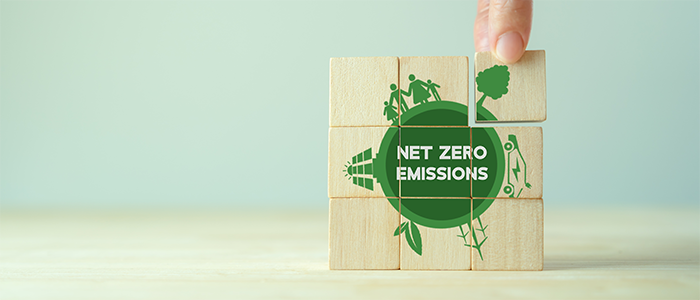
EVs – a brand’s catalyst towards Net Zero goals
EVs – a brand’s catalyst towards Net Zero goals
The need for sustainability to co-exist with commerce is more imperative than ever, as evident from the rising carbon emissions. With the world marching towards net-zero goals and businesses cementing their efforts to reduce their carbon footprint, electric vehicles have emerged as a critical element in a brand’s journey toward zero emissions.

Vivek Nair
Head Brand Strategy and Marketing
Sources of Information:
- https://www.axios.com/2019/05/13/carbon-dioxide-concentration-earth-all-time-high
- https://climate.nasa.gov/climate_resources/7/graphic-carbon-dioxide-hits-new-high/
- https://newsroom.accenture.com/news/nearly-all-companies-will-miss-net-zero-goals-without-at-least-doubling-rate-of-carbon-emissions-reductions-by-2030-accenture-report-finds.htm#:~:text=1%2C%202022%20%E2%80%93%20While%20more%20than,Accenture%20(NYSE%3A%20ACN).
- https://www.eqmagpro.com/net-zero-many-india-inc-majors-could-be-net-zero-by-2050/#:~:text=More%20than%2030%20Indian%20companies,emissions%20by%202050%20or%20sooner.
- https://economictimes.indiatimes.com/news/international/world-news/only-29-of-the-top-50-firms-have-time-bound-carbon-neutrality-targets-report/articleshow/90916911.cms
- https://www.aboutamazon.com/news/sustainability/20-more-companies-from-around-the-globe-join-the-climate-pledge
- https://timesofindia.indiatimes.com/business/india-business/24-companies-including-tata-ril-pledge-to-be-carbon-neutral/articleshow/79072359.cms
- https://www.epa.gov/climateleadership/scope-1-and-scope-2-inventory-guidance#:~:text=Scope%201%20emissions%20are%20direct,boilers%2C%20furnaces%2C%20vehicles).
- https://economictimes.indiatimes.com/industry/renewables/cipla-adds-capacity-of-captive-renewable-energy-power-plant-in-maharashtra-karnataka/articleshow/91955693.cms?utm_source=contentofinterest&utm_medium=text&utm_campaign=cppst
- https://gailonline.com/RenewableEnergy.html
- https://renewablewatch.in/2022/09/13/hindalco-commissions-25-mw-captive-solar-plant-in-madhya-pradesh/
- https://www.electricalindia.in/hindustan-zincs-captive-power-plants-win-award/
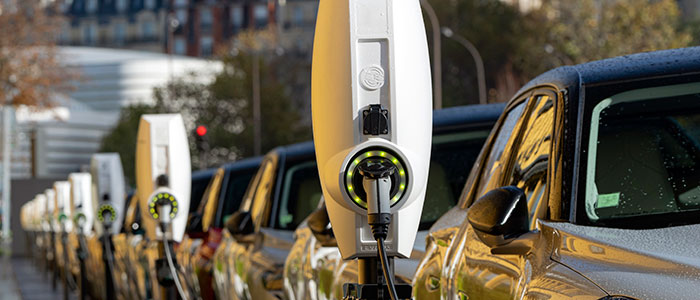
Electric Vehicles and The Innovation Landscape
Electric Vehicles and The Innovation Landscape
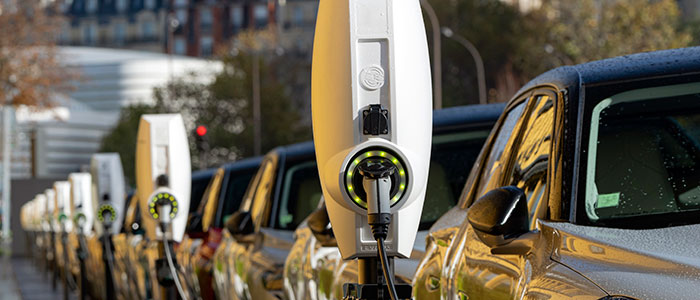
While the fascination with electric vehicles seems to be rather new, it is interesting to note that the first wave of serious investments in the electric vehicle space happened in the early 19th and 20th Century. The excitement with EVs was such that, in early 1900s close to 30% of the vehicles on road were electrically powered as compared to ~20% that were powered by gasoline. However, with increased efficiency gains with combustion engines, greater access to gasoline, and Ford’s improved commercial production, electric vehicles saw a gradual decline from the market to a point where they were totally replaced by ICE vehicles.
Electric vehicles once again caught everyone’s fancy with the remarkable work of material scientists such as John Goodenough, Koichi Mizushima, Rachid Yazami, Akria Yoshino (filed the first patent on a commercial Li-ion battery) in stabilizing the Lithium-ion chemistry for energy storage. This pioneering work led to renewed focus on research in electric vehicles. Such has been the focus on the technology that the number of electric vehicles has risen from just about 150,000 in 2012 to a staggering 6 Million+ in 2021.
With steady advancement in the foundational building blocks of electric vehicles, the world has also seen newer research areas spring up, that are aimed at making the life of an EV user/owner a lot less daunting.
Over the last 20 years, R&D activity around electric vehicles has primarily been focused around the following key categories:
- Battery Systems (IPC Class: H01M and other related classes)
- Propulsion Systems (IPC Class: B60L and other related classes)
- Chassis Control (IPC Class: B62D and other related classes)
- Other Control Systems and Communication Technologies (IPC Class: G05 and other related classes)
Intellectual Property, a significant manifestation of R&D activity, is a great enabler for companies to protect their contribution to a domain for commercial gains. Approximately 4 Million Patents are filed every year across the world that address all of the technological advancements made in that year. More specifically, when it comes to electric vehicles, a simple search of patent applications filed in the last 20 years across different domains shows that more than 85,000 patent applications have been filed in the US, China, Korea, Japan, Europe, and India.
As is evident from the chart above, a large part of activity is concentrated around Propulsion Systems and Battery Systems. The majority of filings in propulsion systems come from established players with 100s of years of experience in Propulsion of traditional vehicles. Whereas, when it comes to battery systems, a large concentration of the portfolio is cornered by chemical giants.
From a geographic standpoint, R&D activity seems to originate in China, followed by the United States based on an analysis of the country of origin of lead inventors for the patents analysed.
Log9 | Patent Portfolio
Our patent portfolio is a manifestation of our ground-up approach towards building customized technologies. We have always challenged the status quo and it is with this mindset that we started innovating at every stage and developed unique and cutting-edge technological solutions.
Log9’s R&D activity focuses on building cell technology and other communication techniques that provide the safest, most reliant battery packs suitable for the Indian Market. With a clear understanding of our role in building an indigenous Li-ion solution for our environment, our team comprising of energetic material scientists and engineers have set their eyes on contributing to research activities in Bucket 1 and 4 identified above. In this year (2022) alone we have moved from identifying the right mixture of chemicals required for a safe battery pack to improving the manufacturing methods and processes that will make large scale cell manufacturing in India a very real possibility in days to come. The result of our work has led to filing of more than 15 patent applications across the world specifically targeted towards battery systems and is one of the most important components of our portfolio of 70 patent applications. We are extremely proud of being the only company in India that has moved from solving the chemistry problem to solving the manufacturing problem. Log9 is further envisaging close to 25 cell manufacturing patents by 2023.
While there is still a long way to go for India to make mark on the global patent map, the roadmap ahead looks positive with a series of innovation in the nation’s R&D ecosystem.
Shailesh Tavate
Head of Intellectual Property
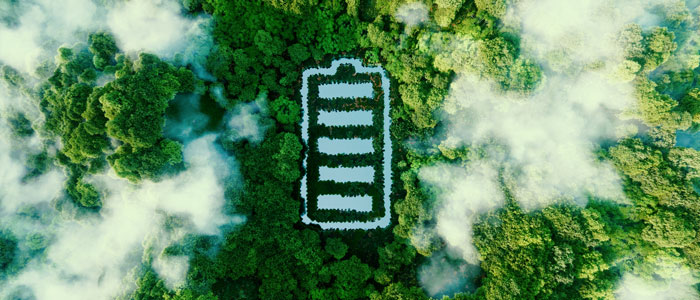
The EV Battery Afterlife: Implications on the Climate
The EV Battery Afterlife: Implications on the Climate
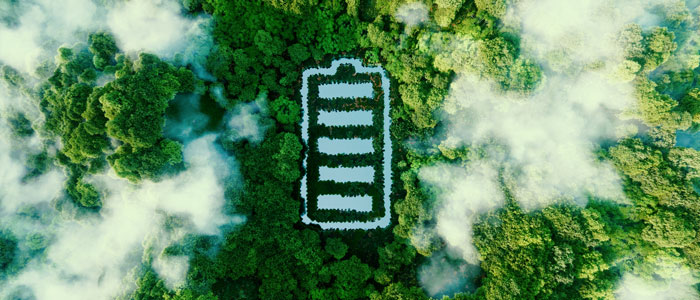
How is the EV industry gaining momentum as a medium to realize the net zero emission target worldwide?
The fight against climate change is more stringent today than ever. With the transportation sector contributing to >16% of the overall global carbon footprint, electric vehicles are proving to be a viable and promising alternative to enable net zero emission targets.
The past 5 years have seen a tremendous spike in EV sales across India and the world alike. Globally, the year 2021-22 witnessed an annual sale of 6.8 million vehicles, while India witnessed an overall sales volume of 0.3 million across vehicle platforms.
The EV sale in India rose by 168% from 2021 and is poised to further witness a CAGR of >40% over 2023-2030. While EVs are being popularly touted as the potent means to reduce carbon emissions, are we cognizant of the silent impact of EV batteries on the overall ecosystem?
By the year 2030, >40 million EVs are expected to be sold in India. Considering the average age of an EV battery being <5 years, we are staring at a possibility of >9 million batteries hitting the landfill by 2030. Taking this scenario into account, a question we must answer now is whether we are prepared to tackle the impending challenges. Have we thought about the right means and ways to recycle Li-Ion batteries? And are we vigilant of the ill impact should we fail to find an answer?
The proliferating demand for Li-ion batteries
Among the various types of batteries available in the market such as lithium-ion batteries, solid-state batteries, nickel metal hydride batteries, lead acid batteries, and ultracapacitors, lithium-ion batteries are the most widely used in EVs today, driven by their high energy per unit mass, high power-to-weight ratio, high energy efficiency, high-temperature performance, and low self-discharge.
The cumulative stock of lithium-ion batteries in India is approximately ~22GWh with applications spread across electronics, stationary, and transportation sectors. The growing trend of electrification across the nation is expected to further push the demand for batteries in the coming years.
The Battery Afterlife
Implications, Challenges, and Roadmap Ahead
While it can’t be argued that EVs are an important component of the net-zero emission targets globally, the challenges imposed on the environment once the batteries run their life course are something that demands immediate consideration to stop irreversible damage ecologically and economically.
What is battery afterlife?
The end of life for Li-ion batteries is a subject of debate and can be broadly put under two categories.
- Traction batteries, ones that allow electric vehicles to run, are considered unfit post losing 20% of their rated capacity, primarily because they don’t meet the range requirements
- Stationary storage batteries typically run till they lose 30-40% of rated capacity
Depending on the use profile of the batteries, they typically last for 5-15 years and still contain >60% of capacity after their end of life, containing a significant amount of critical battery materials.
Li-ion Battery Chemistry
The underlying chemistry of Li-ion batteries defines their performance characteristics. While there have been several cell chemistries introduced over the past decade, the most widely acceptable chemistries today are lithium nickel manganese cobalt oxide (NMC), lithium iron phosphate (LFP), and Lithium Titanate Oxide (LTO).
Here’s a detailed overview of the three chemistries:
Lithium Nickel Manganese Cobalt Oxide (NMC)
In the NMC chemistry, the cathode is made up of a combination of nickel, manganese, and cobalt. Though the material ratio varies for different manufacturers, the common combination is 60% Nickle, 20% Manganese, and 20% Cobalt. The NMC batteries despite having high-specific energy have low stability when compared to other chemistries.
Lithium Iron Phosphate (LFP)
In LFP chemistry, iron phosphate is used as the cathode material and the anode is made up of graphite combined with a metallic current collector grid. The LFP batteries have excellent thermal stability and enhanced safety.
Lithium Titanate Oxide (LTO)
In the LTO chemistry, the anode is made up of lithium-titanate nanocrystals, enabling the electrons to enter and exit the anode very quickly, in turn, making the LTO batteries one of the fastest-charging in the industry. With high thermal stability and safety, the LTO batteries have huge potential in EVs, military, and aerospace industries.
Battery Afterlife | Ecological Implication
With no proper recycling infrastructure in place at present, approximately 9 million batteries are expected to hit landfill in India by 2030. The current commercial Li-ion batteries contain transition metal oxides or phosphates, aluminium, copper, graphite, organic electrolytes containing harmful lithium salts, and other chemicals, which if end up in landfill can pose a grave threat to human health and the overall environment. Moreover, it is extremely complex and expensive to clean up the leaked chemicals, causing both the environment as well as the economy to pay a heavy price for the irresponsible battery disposal.
Battery Afterlife | Economical Implication
For a country like India where there is a dearth of battery-grade materials, the dumping of batteries signifies a massive opportunity and economic loss. With significant capital spent on raw material outsourcing, the country undergoes a huge loss against each battery being dumped without extracting the maximum potential out of it.
The batteries even at the end of their life have more than 50% of their capacity, which implies, that for each battery hitting the landfill, there is massive wastage in terms of raw materials. This further creates a hindrance in the nation’s vision of becoming self-reliant for its energy needs and increases India’s dependence on other countries for its EV tech requirements.
Recycling
The importance of battery recycling cannot be emphasized enough, both from an environmental and economical perspective. However, the recycling of waste li-ion batteries is a highly cost as well as energy-intensive process.
The lithium-ion batteries are subjected to a variety of processes before they are put to recycling due to their complex structure. The batteries are classified and pre-treated through a series of processes such as discharge or inactivation, disassembly, and separation before putting in for recycling.
Two major chemical processes are being deployed for the leaching, separation, extraction, and electrochemical precipitation of li-ion batteries currently on a global scale.
Hydrometallurgical Process
Playing an essential role in extracting rare and strategic metals, the hydrometallurgical process is used in extracting metals in ion form from the black mass in successive steps such as leaching, purification, and recovery of targeted metal by selective precipitation.
Pyrometallurgy
A highly energy-intensive process, pyrometallurgy is a heat-based extraction and purification process in which the Li-ion batteries are heated at a temperature range of between 1200°C and 1600°C. The process basically includes three steps – roasting, smelting, and refining.
Though the current battery recycling infrastructure in India is not viable from the economical as well as technological perspective, it is crucial to ramp up our efforts in this direction to create a circular loop for critical battery materials and ensure resource efficiency.
Roadmap Ahead Toward Realizing an Energy-Self-Reliant India
For India to become energy self-reliant, it is critically important to facilitate an advanced infrastructure for end-of-life battery management. While there are developments taking place on this front, it may take more than a decade for the nation to be well-equipped with a proper recycling setup.
The next question to answer is what can be done to slow down the damage, if not completely stop it, during this timeframe.
Repurposing
Repurposing implies putting the battery to more than one application, thus increasing its utility, and extending its operational time where they contribute towards a green environment. For example, an EV battery after reaching 20% in the traction stage can be put into another application such as stationary storage, wherein the battery could perform efficiently with the remaining capacity after first use. Taking this approach would extend the life of the battery from an application perspective and churn out the maximum potential of the battery before it reaches its saturation.
For an organization aiming to achieve Net Zero, this opportunity for repurposing the batteries would add an extra dimension towards reducing their scope 3 emissions. Scope 3 emissions arise from the activities of assets not owned or controlled by the reporting organization, but that the organization affects in its value chain indirectly.
Approximately 75% of the emissions are generated from the upstream and downstream activities of the company’s value chain, making Scope 3 emissions a critical opportunity toward making a positive difference to the company’s carbon emissions. Some of the 15 scope 3 emissions include “transportation and distribution” along the upstream and downstream value chain, business travel, outsourced employee commute infrastructure, and personal commute by employees.
Given current grid emission factors, the carbon footprint generated by an EV is 30% less than an ICE vehicle. However, if the same EV is powered by renewables, the vehicle’s carbon footprint can go down by more than 90%. On an average, assuming an annual runtime of > 40,000 km, the absolute annual emissions of a 2W, 3W, and 4W, can be brought down to 2 tonnes, 5 tonnes, and 7.3 tonnes, respectively, should the electric vehicles be powered through renewable sources.
With technological innovations in the EV battery space, today’s batteries can have a life span of more than 15 years. Considering a typical EV life of 5-6 years, the batteries can be repurposed for secondary EV applications and then toward tertiary stationary storage applications, augmenting the potential of renewable energy sources such as windmills or solar panels while increasing grid flexibility, stability, and operating economics. By utilising erstwhile EV batteries, brands may enable their net-zero transition while being economically viable and reducing their reliance on the traditional grid.
Extending the life span of batteries
Repurposing batteries is only viable if the overall lifespan of the batteries is amplified. This approach would help the nation with a time-bandwidth to build a fully-fledged recycling process. The longer battery life would stretch the saturation period of the batteries enabling their multiple uses, thereby extending the period by which the batteries would be hitting the landfill.
Log9 | Pioneering solutions for the future
Marching with the motto of Pioneering Responsible Energy, Log9 is always at the forefront of the fight against climate change. To realize the word ‘sustainability’ for the electric mobility space, in entirety, we fine-tuned our technology to deliver the perfect balance between conscience and commerce.
Our RapidX range of batteries, with LTO technology at its core, have a lifespan of more than 15 years and is one of the safest and fastest-charging in the Li-ion battery category.
The long life of Log9’s RapidX batteries enables them to be used for a minimum of 2-3 applications. For instance, after finishing its first life applications and hitting 20% of its rated capacity while deployed in a 3W, the batteries can be utilized in stationary storage applications such as a telecom tower which requires lesser DOD and can run efficiently with the remaining capacity. The multiple application scope enables churning out maximum potential against each battery.
Though the Li-ion battery recycling infrastructure is at a nascent stage at present, there are massive efforts, both in the public as well as private sectors, to equip the nation with a fully-fledged, technologically advanced recycling setup. As per a report by JMK Research, recycling of li-ion batteries will create a USD 1 billion opportunity in India by 2030. Needless to say, there would be a whole new dimension added to the energy self-reliance vision of the nation upon the realization of a localized recycling infrastructure.
Shikha Sinha
Manager - Content Strategy and Development
Rajat Singh
Sustainability & Stationary Energy Storage - Founders Office
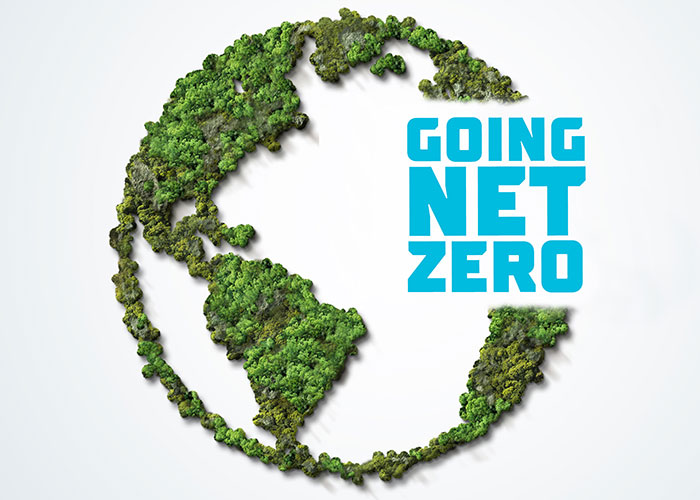
Energy Conundrum – Not a Unilateral Problem
Energy Conundrum – Not a Unilateral Problem
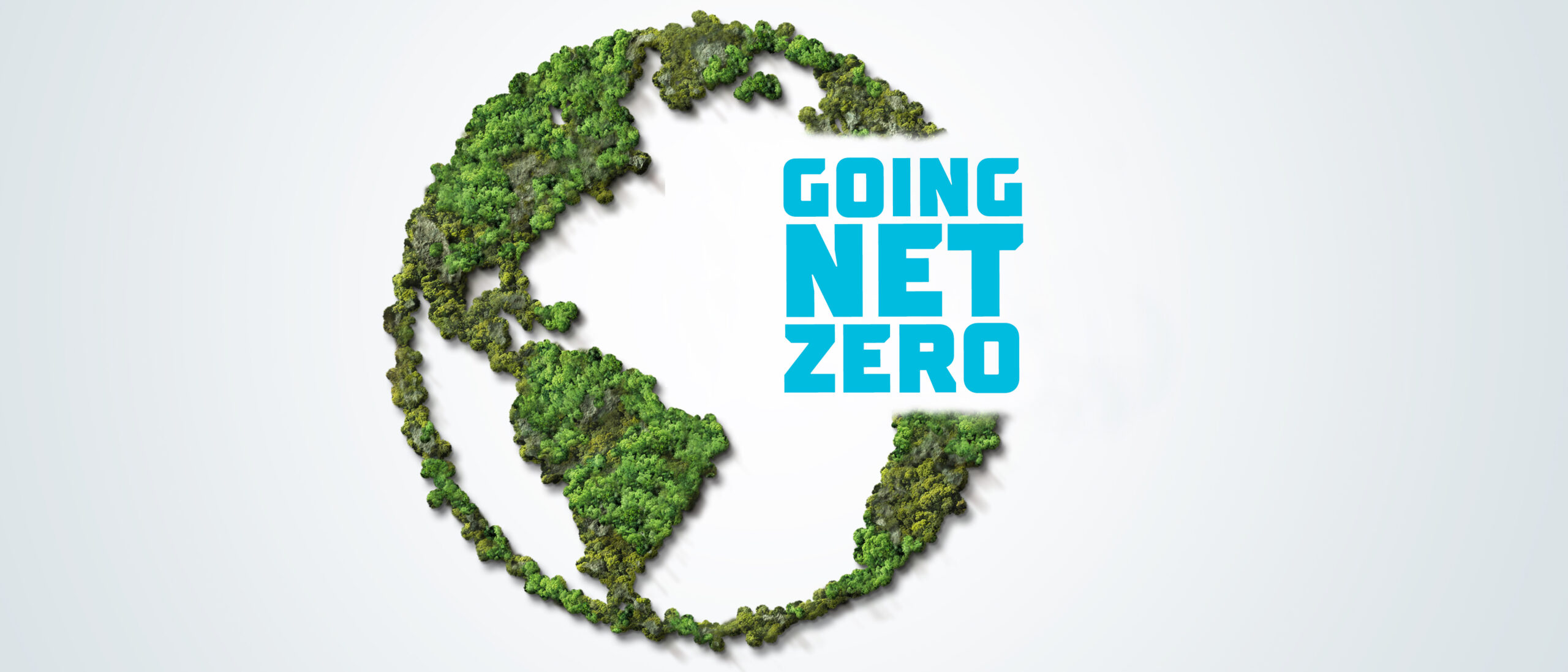
The government of India is taking huge steps toward achieving COP26 milestones – to reduce the overall carbon emissions by one billion tonnes and meet 50% of its energy requirements from renewable energy by 2030. According to a report, the electricity and heat production sectors contribute to 25% of the global GHG emissions.
In India, the thermal energy production is the biggest contributor (41%) to GHG emissions. The current energy landscape of the country reflects an energy mix of 59% fossil fuels and 41% of oil and renewable sources, such as wind, solar, small hydropower, etc.
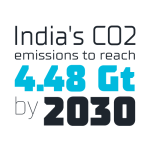
As per a CSE report, the India’s CO2 emissions (as of 2021) are 2.88 gigatonne (Gt). Considering the increasing energy demands, if the energy mix continues as usual, the emissions will reach to 4.48 Gt by 2030, only to alleviate the adversities to the environment, such as air pollution, greenhouse gas emissions, overconsumption of natural resources, and waste generation.
The substantial increase in the consumption of thermal power will delay our efforts to meet the climate goals. To reduce the thermal power consumption for energy production, it is imperative to increase the RE resources. If no damage control measures are adopted, the situation will worsen with the consistently rising amount of carbon footprints.
We can win the fight against climate change by making collective efforts toward augmenting the RE capacity to address the current energy conundrum. Reversing the damage is the need of the hour to bring the planet back to how it was before we brought upon it a climate change havoc.
If not now, when? It’s true that we are looking at ambitious targets to reduce the GHG emissions. But is this enough?
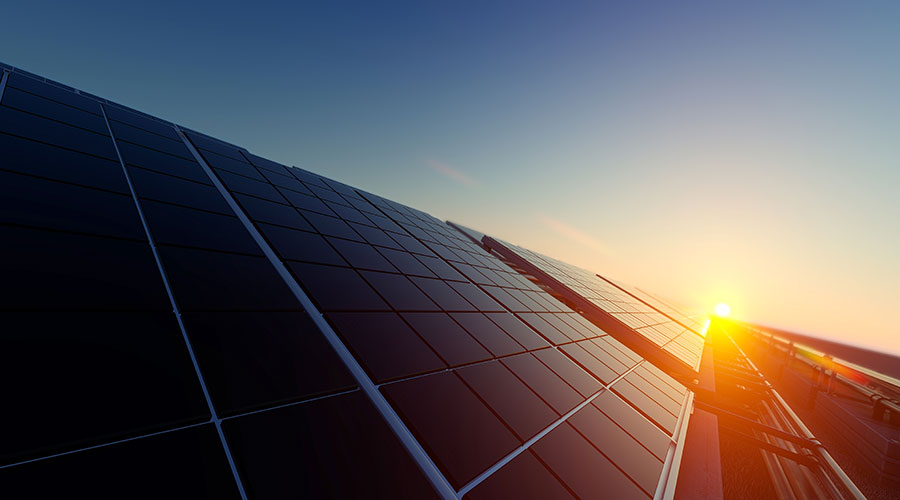
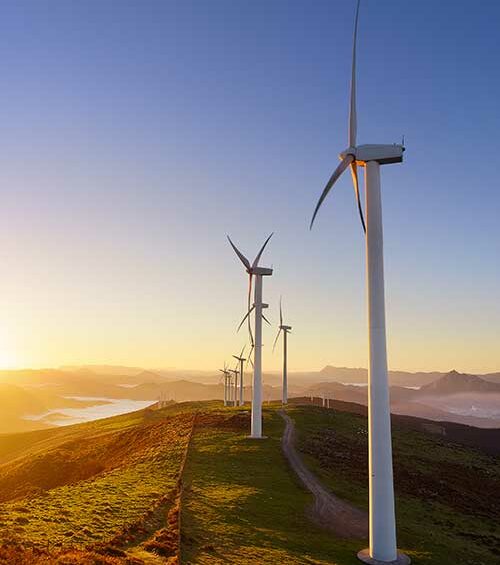
India’s goal to achieve net-zero emissions
India is looking forward to achieving net-zero emissions by 2070. The government realizes that to achieve net-zero emission in the next six decades, we need to meet the rising energy demands with low-carbon energy sources, which require higher RE resources.
What do net-zero emissions entail? Net-zero emissions mean cutting down the net GHGs emissions from sources and sinks to zero. Realizing this goal will enable India to have rich resources of renewable energy by removing an equivalent amount of GHGs from the atmosphere and storing it in plants, soil, or materials.
India has brought about revolutionary changes in the power sector, progressing to reduce our share of power generation from fossil fuels. On the Renewable Energy (RE) side, India has projected a target of producing 175 gigawatts (GW) of RE by 2022 – 100GW from solar, 60GW from wind, 10GW from biomass energy, and 5GW from small hydropower. Based on the announcement by the Union Ministry of New and Renewable Energy, we installed 100GW of RE capacity, which extended the target to 500GW by 2030.
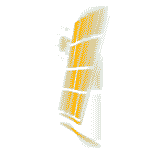
However, with the country’s increasing GDP and population, the primary energy demand has increased and will continue to increase in the next decade. Electricity consumption has grown at a CAGR of 7.39% and electricity demand is expected to reach 1900Terawatt-hours (TWh) in 2030.
Despite all these efforts and bold promises, we stand as the third-largest emitter of GHGs across the world. This justifies the urgency of implementing RE as an alternative to fossil fuels for meeting higher energy requirements. Along with our efforts to increase RE resources, we must also look for energy-efficient measures to lower our overall energy consumption. But, is it that simple? Let’s dig deeper to understand better.
According to various studies, the most abundant renewable sources are sunlight and wind which can reduce fossil fuel consumption significantly and ultimately CO2 emissions. There are several challenges to ensuring the utilization of available renewable energy sources.
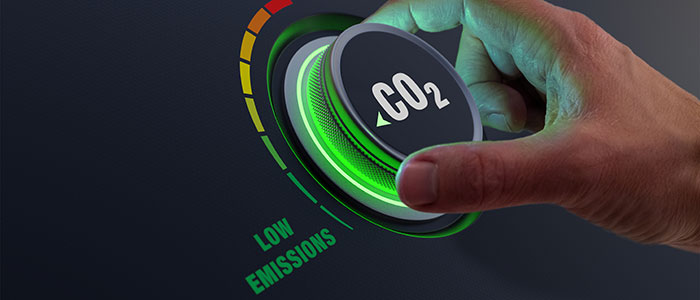
Challenges to address for achieving net-zero emissions
Intermittent power supply:
Solar/wind energy sources are intermittent which means that they cannot produce energy every hour of the day as they are dependent upon the climatic conditions. India experiences a tropical climate, which exposes us to abundant sunlight to generate solar energy. But, are we able to generate the estimated capacity of solar or wind energy? Due to its erratic nature, it becomes difficult to utilize optimum amount of sunlight. Wind energy also involves the unpredictability of wind flow. It is a vital problem with renewable energy and its intermittency, which presents new challenges for effective grid management and operations.
To resolve this, renewable energy should be assisted with advanced and efficient energy storage solutions, and Li-ion batteries are the most desirable energy storage devices today. Here’s another challenge – our dependence outside India for getting these Li-ion batteries.
Dependency outside India for raw materials and components for solar panels:
Although we have a strong manufacturing base for wind energy equipment, ~87% of our solar equipment is sourced from outside India. This makes us highly energy-dependent and increases the overall cost of RE production.
Lack of infrastructure for efficient Transmission and Distribution (T&D) of the generated power supply:
Nearly a quarter of electricity generated is lost in transmission because India’s distribution companies are using outdated infrastructure, resulting in line faults and leakages, as well as undersized and over-utilized transformers. Moreover, weak monitoring and sloppy maintenance standards lead to frequent power theft. We need to have more accurate systems to monitor the T&D of the generated power.
Lack of energy efficiency:
Due to the low energy density of renewable energy sources and other technical issues, it becomes challenging to meet the increasing energy requirements and reduce the GHG emission to the net-zero level alone.
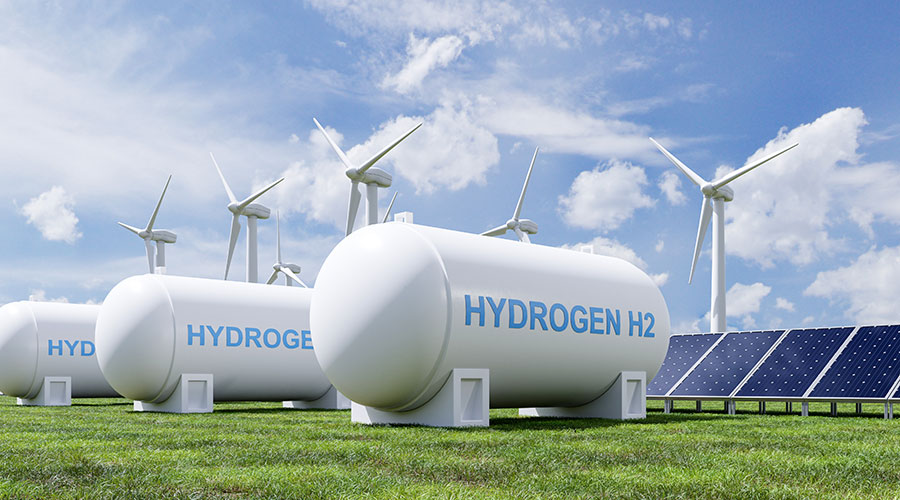
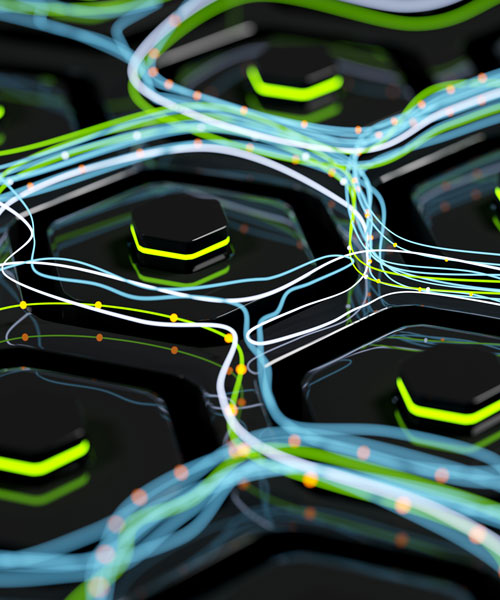
Plugging in the gaps to meet the RE and GHG emissions targets
Develop energy storage devices
Due to the intermittency of solar/wind energy, it is significant to have indigenous cutting-edge energy storage devices. These devices can store energy when sunlight and wind are abundant to enable their usage during their absence. Energy storage devices also serve the purpose of the much required grid ancillary services for smooth grid operations, currently met by conventional synchronous plants. Not just this, the hybridization of solar/wind energy using energy storage devices will provide us with a sustainable solution, reducing the dependence on the grids.
So, what are the best energy storage devices? We will need high-power Li-ion batteries to offer energy storage and power solutions. In the current scenario, India imports Li-ion batteries in large quantities to fulfil its energy storage needs. But it only makes us energy-dependent and energy storage turns into a costly affair. So, deploying battery packs with RE-based systems becomes challenging.
Develop indigenous and sustainable battery solutions
To become energy sufficient and use RE for energy storage, it is significant to develop indigenous and sustainable battery solutions. The batteries made in India will be best suited for the diverse climatic conditions, decreasing not only the cost of operations but ensuring battery safety as well.
Increase investment in fuel cells
To meet the consistently increasing energy demands, India must increase the investment in other renewable sources like fuel cells (e.g., Hydrogen fuel cells and Aluminium Air batteries) in addition to solar/wind energy.
Hydrogen fuel cells are one of the best renewable sources of energy for their 100% clean and high energy efficiency over other fossil fuels. However, it still leaves some of the challenges untapped, such as complex storage, costly production, and safety issues we need to overcome to realize the full potential of Hydrogen.
In this direction, Aluminium-Air Fuel Cells (AFCs) are a promising alternative to fossil fuels and Li-ion batteries because of their theoretically high energy density (20X as compared to state-of-the-art Li-ion Batteries) and solid recyclable fuel. These are primary batteries truly powered by Aluminium and water. Since India is the second-largest producer of Aluminium in the world, AFC technology can make the nation resilient, and save a huge amount on import bills which can be otherwise utilized to strengthen the economy.
Moreover, such fuel cells do not require robust grid infrastructure and make remote and rural areas self-sustaining in their electricity needs.
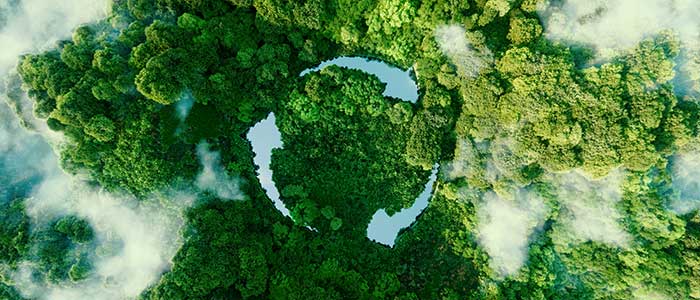
Bridging the competency gaps
According to CEEW (Council for Energy, Environment and Water), one of the major obstacles to realizing India’s net-zero dream is the competency gap. To attain the RE capacity goals, we need 2+ lakhs trained site engineers and 6+ lakhs semi-skilled and low-skilled technicians. In addition to this, we need a cohort of professionally trained individuals in material science, R&D, and deep technology to build innovative energy storage solutions.
According to industry surveys, blockchain, cloud computing, software development, AI and UX designs are the most sought-after skills today. It is high time we also focus on utilizing our young talents from the reputed IITs, NITs, and ITIs across the country. Offering them professional training in modern scientific technology can be an asset for the country to move closer to its sustainability goals. Investing in training the engineers in the renewable energy industry is a fundamental need for a greener future.
Framing policies for stringent safety standards and technology adoption
An end-to-end establishment of a safe and sustainable renewable power system in India can only be possible with stringent safety standard policies. Undoubtedly the government is framing incentive policies to promote the adoption of greener technologies, but it is equally substantial to take necessary action to further increase the awareness about clean energy technologies amongst the Indian population, encouraging mass adoption of clean tech.
Talking about the safety standards, it is imperative to ensure the safe utilization of energy storage solutions (Li-ion batteries). Furthermore, efficient recycling and dumping policies should be in place to prevent the generation of secondary pollutants, such as solar panels and li-ion battery wastes. These materials can be reused, reducing the import of raw materials from foreign countries.
So far, for both onsite and offsite RE projects, the central and state grids provided net-metering and banking benefits, essentially a form of free energy storage, to increase intermittent RE adoption. With new regulations coming in, moving away from net-metering and banking, and moving towards real-time settlement of intermittent RE generation, energy storage policies will have to be baked in by the electricity regulators to increase the BESS economic viability.
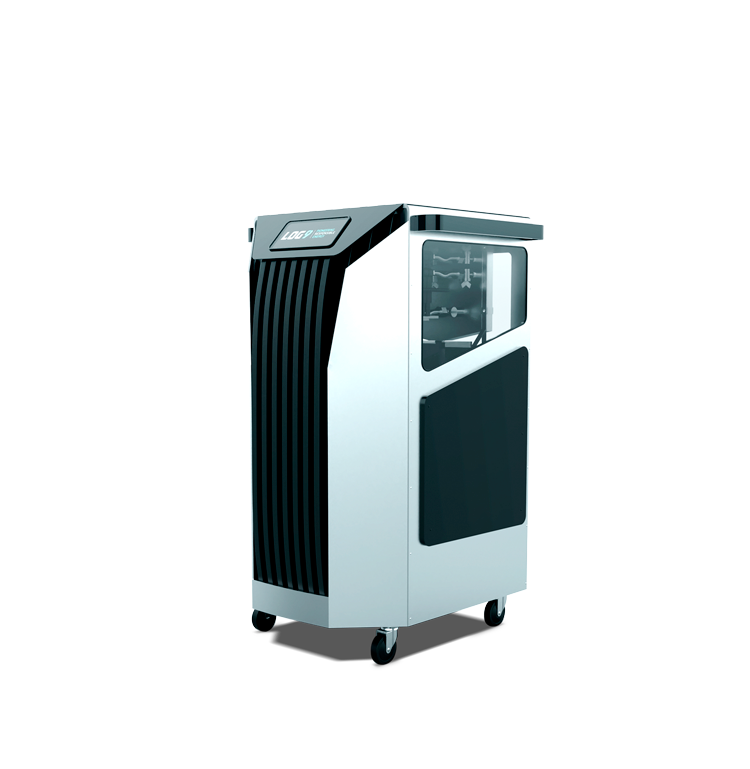
Log9 – Contributing to resolving the energy conundrum
As a pioneer in developing responsible energy, Log9 is investing in sustainable energy storage solutions with a vision to create a greener world to live in. Our advanced Li-ion battery technology promises a life cycle of 15 years. The battery packs are developed considering the tropical climatic conditions, making them suitable for the entire tropical belt.
Being a deep-tech startup with a commitment to revolutionize the Indian EV industry, we come with competencies across materials to cell to pack level fabrications for energy storage solutions. We understand the need for making RE a sustainable option and all our efforts focus on attaining the goal of net-zero emissions.
Going further, our AFC technology will support long-haul EVs and stationary applications. Log9 considers innovation as the cornerstone and continues to pursue it towards making the world sustainable with modern energy storage solutions.
Rajat Singh
Sustainability & Stationary Energy Storage - Founders Office
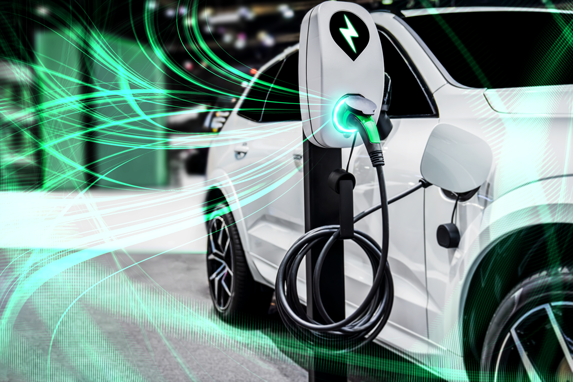
Are Today’s EVs Truly Green?
Are Today’s EVs Truly Green?
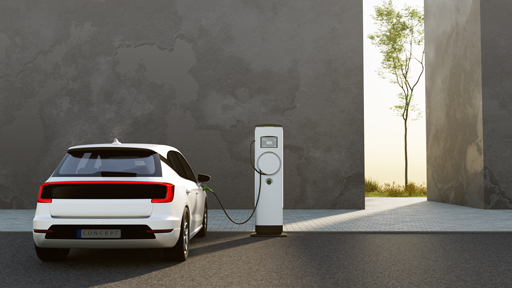
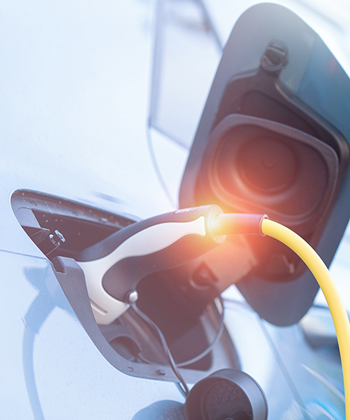
EVs have shown promise globally and it’s no different for India. The Indian EV industry has witnessed dramatic growth over the last three years with a CAGR of 60% from 2019 to 2022 (as per Vahan Portal for battery operated vehicles). A total of 0.32 million EV vehicles were sold across the country in 2021 alone which was the highest in the history of Indian EV industry.
Shri Nitin Jairam Gadkari, the Minister of Road Transport and Highways of India, has projected a target of deploying 3 crores EVs by 2024 and the costs of EVs would match the cost of ICE (Internal Combustion Engine) vehicles. India will become the third largest automobile industry across the globe by 2030 as we meet these targets.
Government bodies like NITI Aayog have introduced policies aiming for 100% electrification by the end of 2030. This increase in affinity toward EV vehicles can be justified by their eco-friendly nature and the consistent rise in fuel prices.
Nevertheless, there are plenty of speculations around the sustainability aspect of EV adoption. Are EVs serving the purpose of fighting climate change? Are EVs truly green?
But before we answer these questions, let’s understand if we are measuring the right metrics.
Are we measuring the right metrics?
To understand this, we need to dive into the concept of measurable metrics relevant to EVs and the process of manufacturing. First things first, let’s talk about a few crucial terms associated with EV manufacturing.
The energy mix is the combination of different energy sources like thermal, solar, wind, fossil fuels, etc. used to meet the energy needs in a geographic region. Today, India has an energy mix of 56.7% coal and the remaining 43.3% accounts for oil and renewable sources like solar, wind, hydro, etc.
Embodied carbon emissions are the total amount of carbon emitted during the manufacturing and production stages of an EV. The main contributor to these emissions is the battery manufactured for EVs which accounts for >50% (as of today) of the total embodied emissions. The gCO2/km is the functional unit used to calculate the carbon emissions released per kilometer.

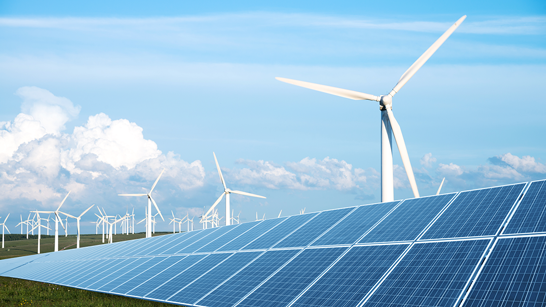
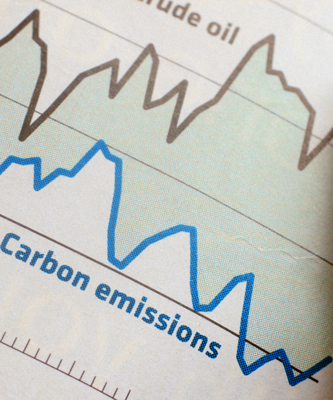
When it comes to measuring the metrics associated with the EV ecosystem, both financial and sustainability metrics must be taken into consideration. While the financial metrics (INR/km) remain the primary measurement metric, it is equally crucial to track the sustainability metrics (gCO2/km).
Sustainability metrics shouldn’t only include the operational emissions (emissions after the EV hits the road), but also the embodied emissions that leave a larger impact on the environment than ever anticipated. Embodied emissions are often overlooked by the end consumers due to a lack of knowledge and awareness. Mostly, the performance of EVs is measured based on their low operational costs (INR/km) and emissions and zero tailpipe emissions after the vehicle hits the road.
Furthermore, we should also look at other essential metrics to calculate the performance of EVs, such as the Total Cost of Ownership (TCO) through the life of the vehicle and the battery life. The total cost of ownership is an overall estimate of the total expense incurred right from the production, purchase, to deployment and entire lifecycle of the electric vehicle.
When we start measuring the right parameters, it broadens our scope of understanding the EV ecosystem from different perspectives.
How is the change in perspective going to affect the current scenario?
Of the many myths around EV adoption, one is net-zero emissions. When a person buys an electric vehicle, the first thought that comes to mind is taking a step toward fighting climate change. While this is partially true, there’s another side of the story – the energy and resources that go into the production process.
The life cycle of an EV goes beyond its total runtime. It starts with the battery manufacturing process and continues through the EV deployment and its operational life. Battery manufacturing lead to high carbon footprints, which takes years to break even with ICE (Internal Combustion Engine) vehicles. The raw materials used, such as lithium, copper, nickel, and manganese emit toxic emissions.
The gaps are not limited to the amount of carbon footprints created during the battery manufacturing process. The energy mix in the national electrical grid is also a responsible factor as the majority of the energy resources are non-green (thermal/coal).
Another significant point of consideration is the life cycle of a battery. The battery life is determined by the cell technology and the # cycles utilized in the use phase. With higher utilization in commercial vehicles, a battery replacement is bound to come that not only increases the Total Cost of Ownership (TCO) through the life of the vehicle, but also emissions accrued to battery manufacturing.
So, buying an EV doesn’t reflect a positive ecological impact instantly, neither does it breaks even with an ICE vehicle. It takes years to reach a parity point after the vehicle has hit the road, Parity point is achieved when the total emissions reduced during the use phase of the vehicle is equivalent to its total embodied emissions.
Now the question is, how do we bridge these gaps? According to sustainability experts, developing clean energy is a responsible step for making EVs greener. Scientists and industry experts are also looking for more efficient battery technologies that ensure lower embodied emissions and a longer life cycle.
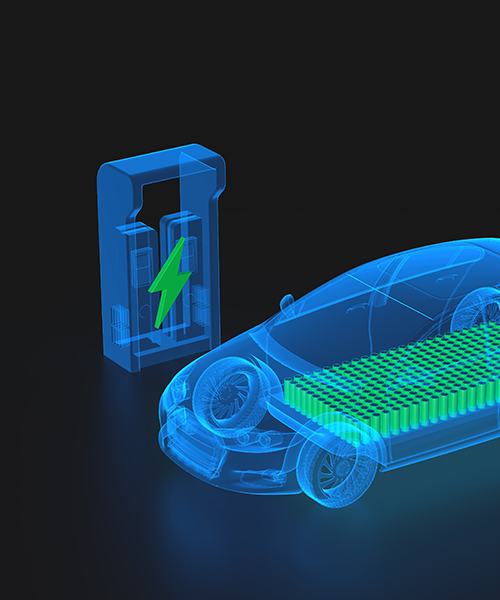
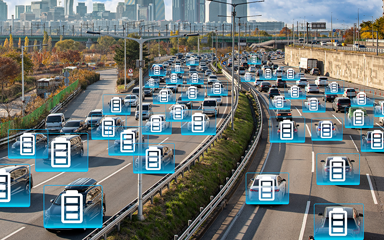
When do EVs become truly green?
Although the operational costs and emissions of EVs are lower than their ICE counterparts, it is essential that the lifetime emissions of electric vehicles break even with a combustion engine vehicle. It happens when an EV reaches the parity point.
After an EV customer reaches the parity point, the vehicle turns truly green. The time it takes to achieve the parity point is called green payback years.
How long does it take to reach the parity point? Let’s evaluate the runtime across the life of the vehicle to understand this.
Typically, a personal user drives for >15kms a day, ~40,000kms over a 5-year life (smaller platforms like 2W), and 80,000-1Lkms over an 8-10 year life (heavier platforms like 4W). Bigger the battery pack, the higher the embodied emissions difference and the higher the parity point and payback years. A simple assessment portrays the following:
The above table shows that a 2W must drive for 15,000kms or >1.5 years to unlock a green parity point, whereas a 4W must drive ~1,00,000kms or >6.5 years to achieve a green parity point. These numbers vary in the case of commercial EVs due to the higher runtime (>100kms a day), reducing the payback years. However, a lower payback period also indicates faster battery degradation and replacement, which ultimately increases the TCO for the consumer, both economically and environmentally.
So, does the battery type used in the EVs lasts for 5 years to reach the parity point? Is there a permanent solution to these new challenges in the EV ecosystem?
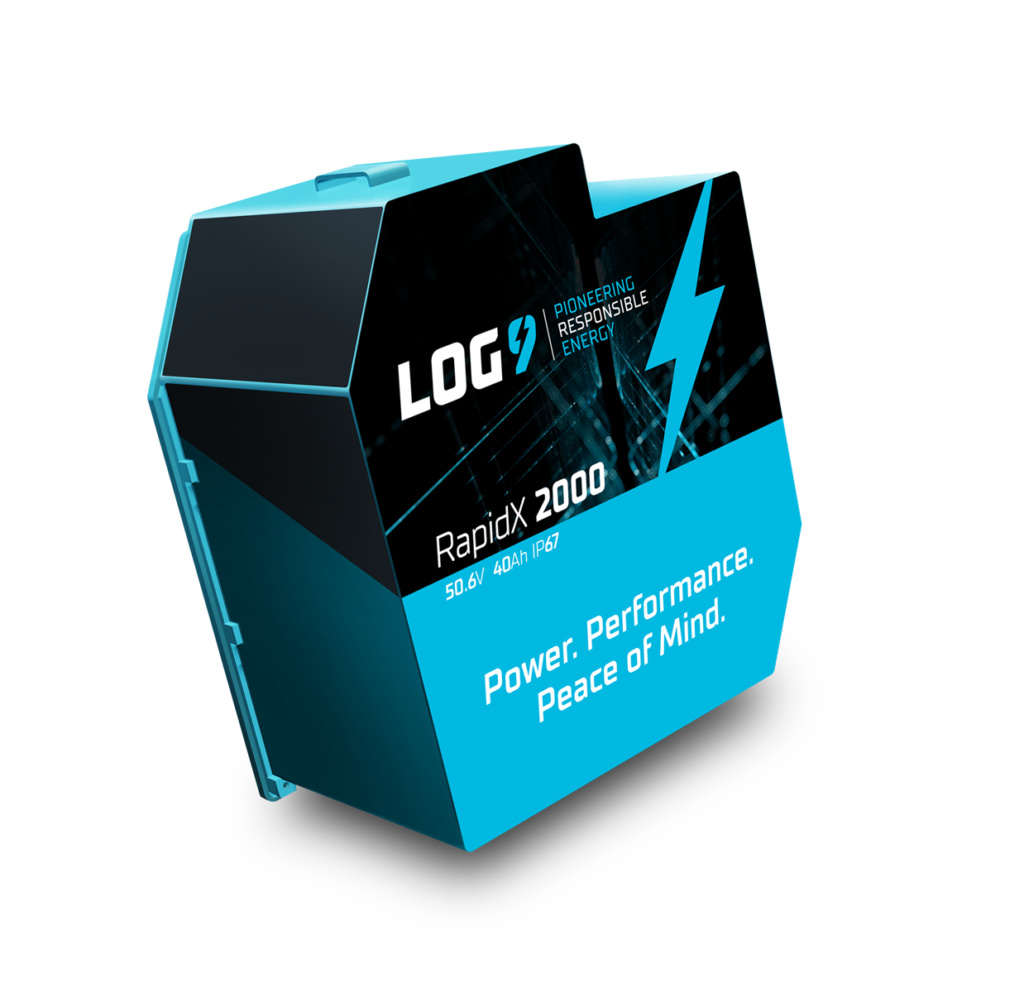
Log9 - Pioneering responsible batteries
To resolve the challenges of mass EV adoption and with an intention to ensure electric vehicles truly become a greener alternative to ICE vehicles, Log9 has come up with its indigenous battery technology.
Log9 batteries, having a high cycle life, allows utilization of each battery for multiple commercial EV applications (>3, minimum 15 years), whereas traditional batteries can power multiple units (~2-3) for just 1 commercial EV application. The longer life cycle of batteries (>10 years) eliminates the need for battery replacement every 3-4 years, ultimately cutting down on the TCO.
While a conventional battery ends up in the landfill after 3-4 years, Log9 batteries continues to deliver high power, performance, and peace of mind to the end users. Through the life of an EV vehicle powered by Log9 batteries, it records a notable reduction in the carbon footprints, creating a positive impact on the environment.
The key to unlocking the true decarbonization potential of the transportation industry and the logistics segment lies in advancements in cell chemistry and material choices that deliver capabilities suited for implementation. Battery technology selection for EV manufacturers will be a significant driver in supplying EVs for e-commerce and logistics service providers in the future.
Rajat Singh
Sustainability & Stationary Energy Storage - Founders Office